Organism with Unnatural DNA
3-25-21
The process of DNA replication in a normal cell begins with the separation of double stranded DNA in an adenine-thymine rich region. The reason for this is because there are only two hydrogen bonds to break instead of the three between guanine and cytosine. DNA-A is the protein that binds to its respective binding region and causes the separation of the two strands. Single stranded binding proteins then bind to the single strands to prevent them from reannealing to each other. When the single strands are exposed, the replication machinery begins to assemble in the region. Helicase comes in and recruits other proteins to the site. Primase comes and binds to the N-terminal of each helicase, DNA-C detaches from helicase and allows it to close on the ssDNA. DNA-A detaches once the two replication forks have the primase-helicase complexes. The primases lay down RNA primers, clamp loader proteins come in and two TauCs connect them to the replisome. The clamp loader loads the clamp that connects DNA polymerase to the ssDNA, replication begins as a leading and lagging strand are built.
Transcription is the creation of mRNA from DNA, the cell must need a certain protein for transcription to occur. To start, it needs a sigma factor to bind RNA polymerase to the promoter. The sigma factor opens the dsDNA and transcription can begin. Transcription is terminated by the mRNA at a guanine-cytosine rich region. Sometimes termination needs a Rho protein. Before translation can occur, the mRNA is processed by removal of introns and connecting the exons. Once the mRNA is mature, it travels through the cytoplasm to a ribosome, where the nucleic acid sequences translate into amino acids. The 5’ cap enters the ribosome first with the start codon and it begins to feed through the ribosome. As it passes through, tRNA brings the respective amino acids and they are bound together into a chain through the ribosome. Once translation is finished, the two subunits of the ribosome release the polypeptide chain and the mRNA disintegrates.
What makes these two newly discovered nucleotides interesting is their lack of hydrogen bonding between each other. It seems that the only thing that keeps these two paired together is how they are hydrophobic to the environment surrounding the DNA. What forces these two artificial nucleotides to pair up despite their lack of attraction to each other is the presence of triphosphate. With triphosphate, polymerase is induced to create a closed conformation, locking the two nonpolar nucleotides together (Betz, 2013). What can come of these new base pairs includes new amino acids which can create new proteins. With new proteins and amino acids to work with, the cells containing these two new nucleotides can potentially have more information storage and new ways of regulating and metabolizing. An expanded number of amino acids can mean new enzymes with new functions, these functions can be extra protective measures to prevent disease-causing errors.
To discover the potential effects and opportunities of these new unnatural nucleotides, an experiment was run to implement it into E. coli bacteria. To achieve this, plasmids are used to harbor the nucleotides similarly to how genetic techniques are used to introduce a new gene and for clone amplification purposes. This method works by digesting plasmids with an enzyme, then adding the lysed plasmids and target genes into the same solution to be ligated with a DNA ligase, this creates the recombinant plasmid containing the wanted gene. When inserted into a plasmid, the plasmid would then be introduced to the media the bacteria are in. From there the bacteria colony will be able to accept, envelope, and begin replicating it. A problem posed by the successful transport of these nucleotides was the lack in triphosphates present. To address this, nucleotide triphosphate transporters were used to get the plasmids accepted. This posed its own complications with decomposition, causing instability in the extracellular media. The bacteria would deem the unnatural nucleotides toxic and reject the plasmid. An edit was made involving potassium phosphate which made the nucleotides more stable for intake by the E. coli. The unnatural base pairs could be successfully inserted when stable in the media and the decomposition of unnatural triphosphates gave enough time for successful incorporation of d5SICS and dNaM.
Evidence to support the intake of the unnatural base pairs seems most apparent with the slowed growth rate in the cultures that had been given the nucleotides. The use of PCR displayed whether the nucleotides were replicated by the bacteria. When investigating, it is made apparent that the nucleotides were successfully replicated with little error, an error rate like that of natural DNA (Malyshev, 2014). This means that the unnatural base pairs were recognized as components of DNA and when in the rare instance, was repaired by polymerase, were replaced by adenine-thymine pairs instead. In the lab report data, it is shown the doubling of the unnatural nucleotide-containing plasmid through time. This displays the replication and retention of the base pairs in the bacteria culture.
The bacteria appeared to successfully harbor the unnatural base pairs for up to 50 hours before a decrease in retention. The unnatural base pairs were eventually repaired and replaced by adenine-thymine pairs or lost by mispairing due to the eventual loss of unnatural triphosphate. The unnatural base pairs seemed to only pose problems in cell growth due to the initial introduction with the triphosphate transporters used to implement them. When unnatural triphosphate supplements were no longer added and slowly depleted, the base pairs essentially “fell out” of the genome with nothing to tether them. The loss of the unnatural base pairs did not have immediate significant effect on the E. coli cells. However, when looking into long term effects of withholding these nucleosides, it shows a more significant decrease in growth rate due to the reactivity of d5SICS and dNaM to photoactivity (Pollum, 2016). This means that the nucleosides can become toxic to the cells when exposed to photo-chemicals and runs the risk of destroying DNA surrounding the nucleosides.
It is apparent that the unnatural nucleotides slowly disappear in the data shown, displaying the dephosphorylation of the nucleotides over a 6-hour span. The bacteria were still able to replicate the base pairs for a period, providing evidence that they can be sustainable as an outlet for new amino acid and protein creation as well as a new genetic storage tool. It has been shown in this experiment that for bacteria to retain the nucleotides, triphosphates must be continuously supplemented to the bacteria, causing a limitation. For the cells to fully sustain these unnatural base pairs, they would need a way to provide triphosphates for itself and have a new “dietary” requirement. Due to this lack of self-sustaining retention over time in this experiment, it is currently unknown what possibilities these new nucleosides can bring to the genome.
Citations
Betz, K. et al. (2013) Structural insights into DNA replication without hydrogen bonds. J Am Chem Soc. DOI: 10.1021/ja409609j (Accessed: 25 March 2021)
Malyshev, D. et al. (2014) A semi-synthetic organism with an expanded genetic alphabet. Nature, Letter DOI: https://doi.org/10.1038/nature13314
Pollum, M. et al. (2016) Unintended Consequences of Expanding the Genetic Alphabet. J Am Chem Soc. DOI: https://doi.org/10.1021/jacs.6b06822
Nucleotide drawings:
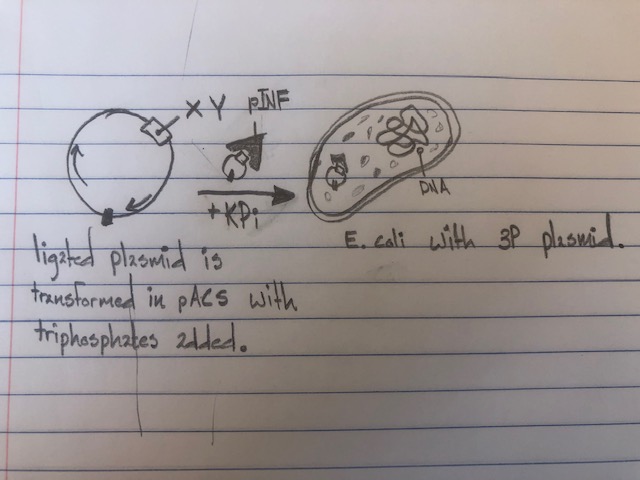
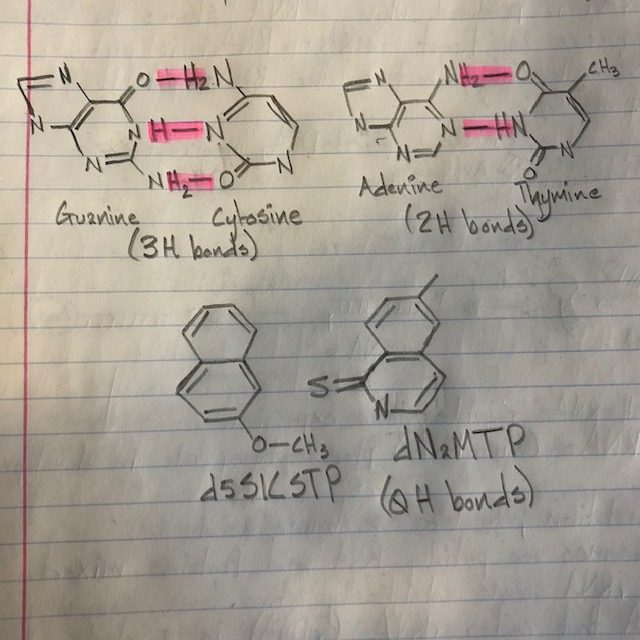