Biomolecule Drawing:
Here is a drawing of a biomolecule I drew for Dr. Steele in my cell biology course!
Cell Drawing
Here is a drawing of a cell I did for Dr. Steel’s cell biology course!
Scientific Literacy
Inorganic Phosphate and its Role on Our Biology
In the world of cellular biology, inorganic phosphate emerges as a silent hero. Often overshadowed by its organic counterparts through its simple chemical structure, inorganic phosphate’s unassuming façade is a world of biological significance in actuality. Represented by the phosphate ion, PO₄³⁻, it is an integral part of the structure and function of key biomolecules within cells, such as the phospholipid layer and our cells’ metabolism. Inorganic phosphate, represented by the phosphate ion (PO₄³⁻), is a fundamental component of cellular biochemistry, integral to the structure and function of key biomolecules such as DNA, RNA, and ATP. As the primary energy carrier in cells, ATP, fueled by inorganic phosphate, drives vital metabolic processes, while phosphate groups attached to proteins regulate cellular signaling and metabolism. Understanding the multifaceted roles of inorganic phosphate provides valuable insights into cellular physiology and underscores its significance in maintaining cellular viability and functionality.
Inorganic phosphates are essential participants in cellular metabolism, fulfilling diverse roles critical for cell function and homeostasis. They serve as central components in ATPsynthesis, facilitating the transfer of phosphate groups from phosphorylated intermediates to adenosine diphosphate (ADP) during oxidative phosphorylation and substrate-level phosphorylation, thereby providing the primary energy source for cellular processes. Moreover, inorganic phosphate acts as a carrier of high-energy phosphate groups in metabolic pathways, participating in phosphorylation reactions that regulate enzyme activity and cellular signaling cascades. Additionally, inorganic phosphates play pivotal roles in nucleotide and nucleic acid synthesis, providing phosphate groups for the formation of DNA and RNA molecules. Through their involvement in these processes, inorganic phosphates contribute to the maintenance of cellular energy balance, biosynthesis of essential molecules, and regulation of cellular signaling pathways, highlighting their indispensable nature in cellular metabolism.
Simultaneously, in the structural domain, inorganic phosphate is integral to the formation and maintenance of the phospholipid bilayer, the barrier that defines cellular boundaries and compartmentalizes cellular organelles. Through its involvement in phospholipid synthesis, inorganic phosphate serves as a substrate for the phosphorylation of glycerol, a key step in the biosynthesis of phospholipids. Once synthesized, phospholipids assemble into bilayers, with their hydrophilic phosphate heads facing outward towards the aqueous environment and hydrophobic fatty acid tails oriented inward. This arrangement forms a stable barrier essential for cellular integrity, regulating the passage of molecules in and out of the cell while providing flexibility and fluidity crucial for various cellular processes.
Multilamellar organelles, also known as multilamellar bodies (MLBs) or multivesicular bodies (MVBs), are specialized vesicular structures found within eukaryotic cells, particularly in the endosomal system. These organelles are characterized by their unique multilayered membrane architecture, which consists of concentrically arranged lipid bilayers. Within multilamellar organelles, small vesicles, known as intraluminal vesicles (ILVs), are formed through invagination of the limiting membrane. These ILVs can encapsulate various cargo molecules, including proteins, lipids, and nucleic acids, thereby segregating them from the cytoplasm and creating distinct microenvironments within the organelle.
The importance of multilamellar organelles to cell function and structure lies in their diverse roles in cellular processes. Firstly, multilamellar organelles serve as key components of the endocytic pathway, facilitating the internalization and sorting of extracellular material. Upon fusion with early endosomes, multilamellar organelles can undergo maturation into late endosomes or MVBs, where cargo molecules destined for degradation are sorted into ILVs and subsequently delivered to lysosomes for degradation. This process, known as endosomal sorting, is crucial for the regulation of cellular homeostasis and the turnover of cellular components. Additionally, multilamellar organelles play essential roles in intracellular trafficking and membrane dynamics, participating in the delivery of proteins and lipids to various cellular compartments and contributing to the maintenance of cellular architecture. Moreover, emerging evidence suggests that multilamellar organelles are involved in intercellular communication processes, including the secretion of signaling molecules via exosomes, highlighting their significance in cell-cell interactions and tissue homeostasis. Overall, multilamellar organelles represent intricately organized structures that contribute to the dynamic and complex nature of eukaryotic cells, playing indispensable roles in cellular function, structure, and communication.
The research investigated how disrupting cellular structures affects PXo bodies, specialized compartments in cells. While some disruptions didn’t impact PXo bodies much, knocking down proteins involved in Golgi protein transport and glycosylation significantly reduced their number, suggesting these processes are crucial for PXo body formation. Additionally, they found PXo bodies primarily originate from newly synthesized phospholipids within the cell, rather than material uptake from outside. Furthermore, computational predictions revealed PXo likely plays a role in transporting phosphate molecules within cells, a finding supported by experiments showing altering PXo levels affected cellular phosphate levels. Overall, the study sheds light on PXo body formation, its role in cellular phosphate regulation, and the structure-function relationship of the PXo protein across different species. As discussed in the walkthrough, when the red and green hues from the dyes are in the same place, it exudes a yellow color, meaning they were in the same place at the same time, the PXo and marker, indicating the structural characteristics of PXo.
The study delved into understanding how PXo bodies, specialized compartments in cells involved in phosphate regulation, respond to changes in phosphate availability and PXo expression. They observed that under conditions of phosphate starvation induced by PFA treatment or knockdown of MFS10, PXo bodies became significantly smaller, while excessive phosphate intake resulted in larger PXo bodies. Furthermore, PXo knockdown led to smaller PXo bodies engulfed by lysosomes, suggesting a role for PXo in maintaining PXo body integrity. Their findings were corroborated by experiments using GFP-labeled PXo, which showed changes in PXo body size and number corresponding to phosphate levels. Additionally, they demonstrated that PXo bodies undergo degradation via lysosomes and autophagosomes following phosphate starvation, a process mediated by the SPX domain of PXo. Overall, the study highlights the dependence of PXo bodies on phosphate availability and PXo expression for their formation and maintenance, shedding light on their role in cellular phosphate regulation.
The study examined PXo bodies, cellular structures involved in phosphate regulation, comparing them to mammalian lamellar bodies found in various cell types. While lamellar bodies are secretory lysosomes formed in the Golgi apparatus and contribute to protective barriers in tissues, PXo bodies show differences in their formation and function. To understand PXo bodies better, researchers developed a method to extract them from fly guts and found they have a higher density than mammalian lamellar bodies. Proteomic analysis revealed unique proteins in PXo bodies that differ from those found in mammalian lamellar bodies, suggesting distinct functions. Further analysis showed enrichment of proteins related to proton pumping, cytoskeleton binding, and metabolism in PXo bodies, highlighting their role in cellular processes beyond phosphate regulation. These findings provide insights into the unique properties and functions of PXo bodies compared to mammalian lamellar bodies.
Figure 4 suggests that the formation and composition of PXo bodies are closely linked to the availability of inorganic phosphates (Pi). When Pi levels are adequate, PXo bodies accumulate phospholipids, particularly phosphatidylcholine (PC), potentially serving as major intracellular deposits for these lipids. Conversely, during short-term Pi starvation, there’s a marked decrease in total phospholipids within PXo bodies, indicating a dynamic response to changes in Pi availability. Stable isotopic tracing experiments demonstrate that Pi consumed by PXo bodies can be converted and stored as phospholipids, highlighting their role in regulating cellular phosphate levels. Overall, these findings suggest that PXo bodies undergo significant changes in composition in response to alterations in Pi availability, underscoring their importance in cellular phosphate regulation.Top of Form
The study aimed to explore the biochemical properties of PXo bodies, specialized cellular structures involved in phosphate regulation. Analysis of the lipid composition of purified PXo bodies revealed a dominance of phospholipids, particularly phosphatidylcholine (PC), which suggests PXo bodies may serve as significant intracellular repositories for phospholipids. Interestingly, changes in phosphate availability led to alterations in the lipidome of PXo bodies, indicating their dynamic response to cellular phosphate levels. Moreover, stable isotopic tracing experiments demonstrated that phosphate consumed by PXo bodies can be converted and stored as phospholipids, with their composition undergoing remodeling in response to phosphate starvation. Additionally, investigation into the signaling mechanisms of phosphate starvation and PXo knockdown revealed interactions between PXo and proteins associated with the Golgi apparatus, vacuolar acidification, and phospholipid metabolism. Notably, PXo was found to interact with components of the Striatin-interacting phosphatase and kinase (STRIPAK) complex, shedding light on potential regulatory pathways involved in PXo function. These findings provide valuable insights into the role of PXo bodies in cellular phosphate regulation and their interaction with key cellular pathways.
Cell Biology Meme
I came up with the idea for this meme after some deep pensive thinking. While in my thoughts, I realized that I had forgotten (once again) to upload my packback assignment for the week, hence, the creation of this meme. It’s a picture of my holding my cat by the neck just like this semester has had on me! 🙂
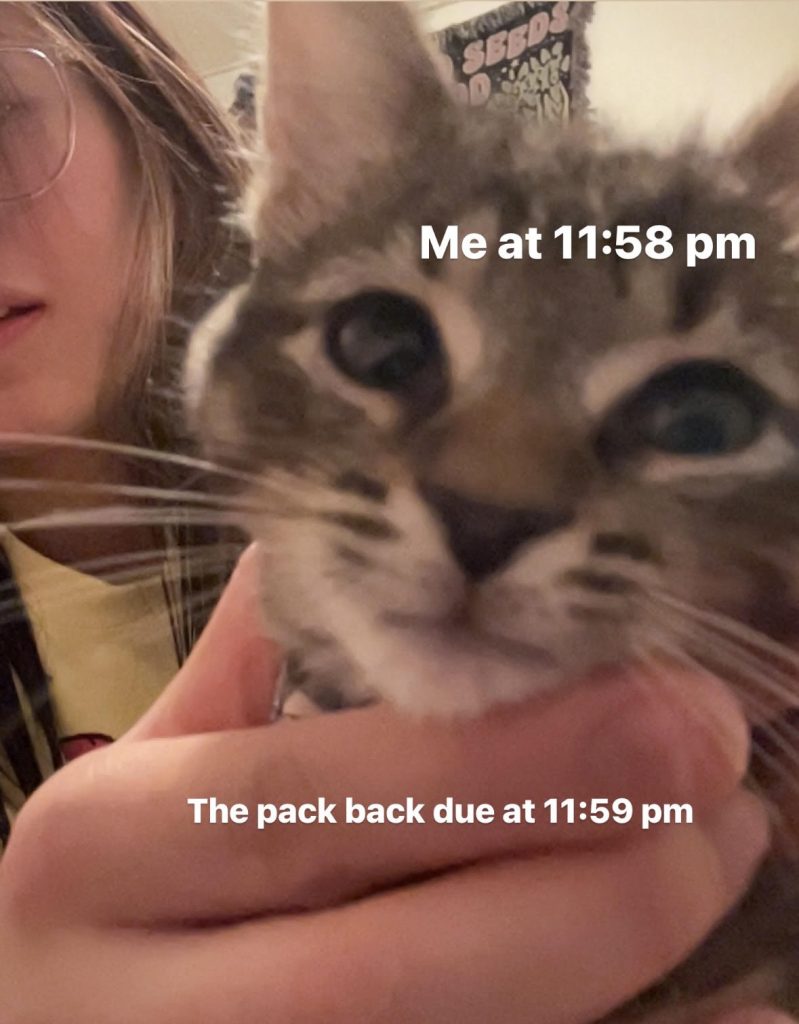
End of Term Reflection
One of the most striking realizations I’ve had throughout this semester is the interconnectedness of cellular processes. Each organelle, molecule, and pathway plays a crucial role in maintaining cellular homeostasis and ensuring the survival of the organism as a whole. From the powerhouse of the cell, the mitochondria, to the intricate network of the endoplasmic reticulum, I’ve come to appreciate the incredible synergy that exists within the cellular environment, which would also include a vaginal microbiome. Abrupt start, but it has helped me with making connections between real life science and my own interest in women’s health and gynecology. Thank you, Dr. Steele (I still can’t remember whether or not your last name as an extra “e” at the end of not)!