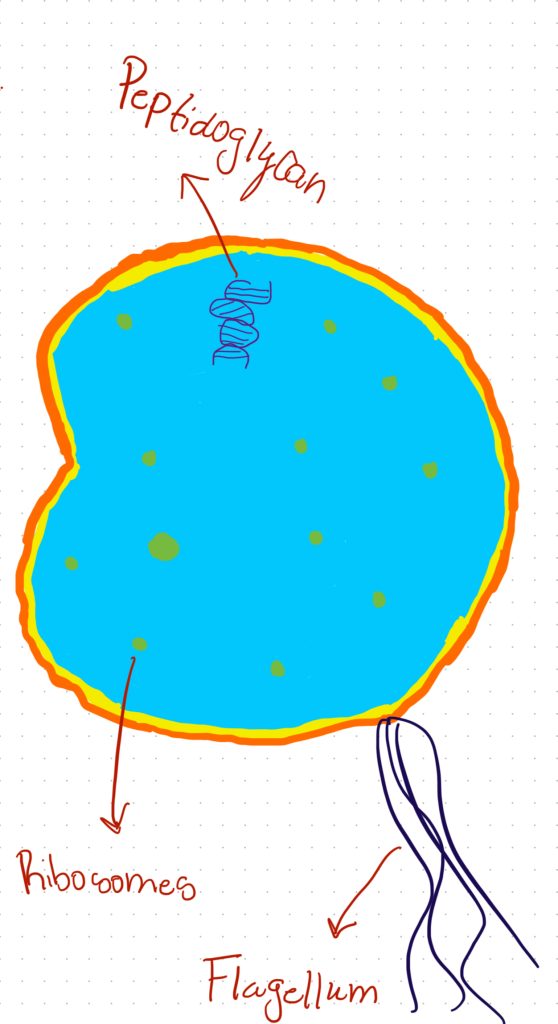
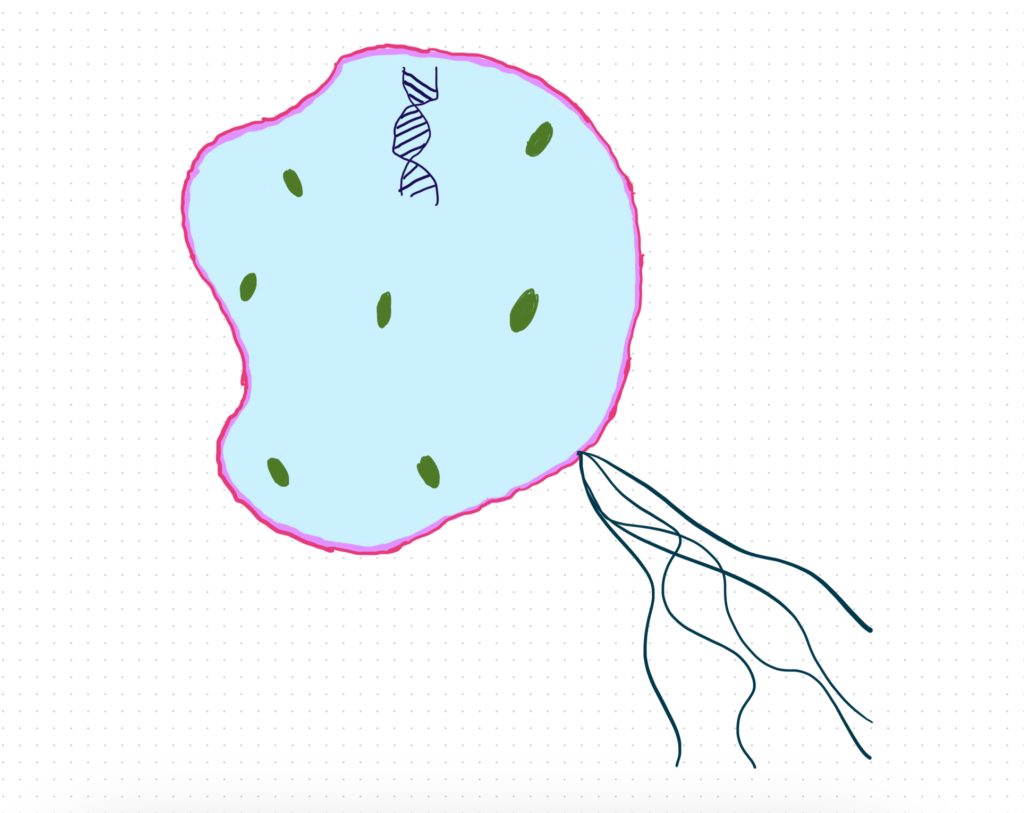
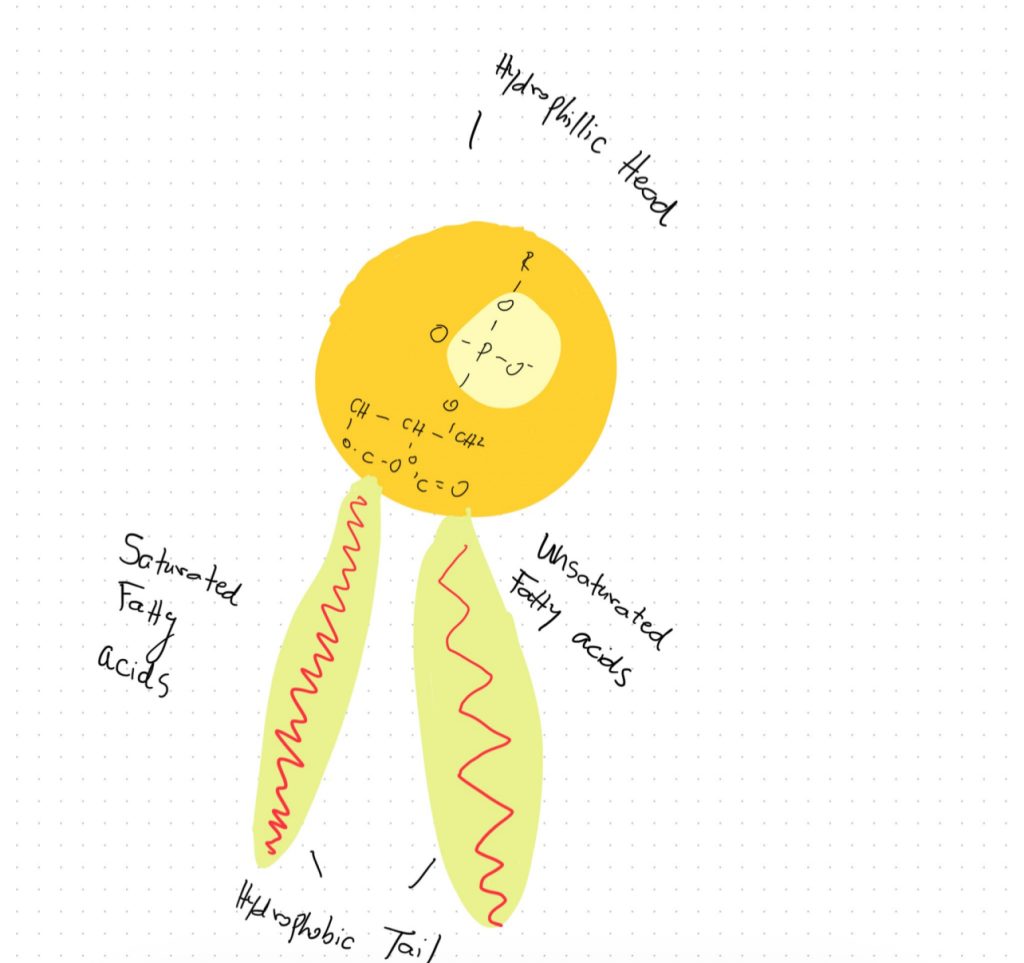
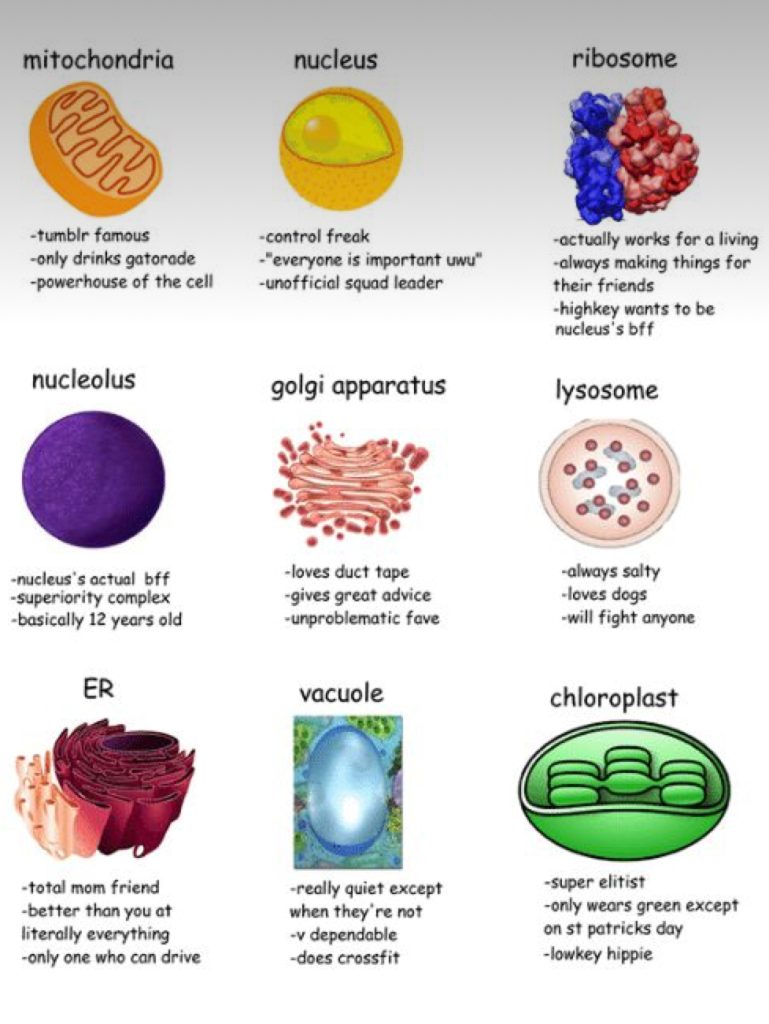
⭐️Scientific Literacy Writings 1&2:
Mia Littlejohn
BIOL 293
Ms. Steel
3-18-24
Introduction to Phospholipid Structure and Function
There are two main aspects of cellular metabolism in which inorganic phosphate is essential. It is first and foremost an essential part of adenosine triphosphate (ATP), the main energy unit in cells. ATP molecules contain high-energy phosphate bonds that are released during energy-requiring metabolic reactions, powering a wide variety of biochemical pathways through the transfer of these bonds (Dunn and Grider). Inorganic phosphate also participates in the biosynthesis of phospholipids, a class of lipids that comprise the majority of biological membranes. Phospholipids are distinguished from other lipids by containing a phosphate group as part of their fundamental structure (Zadoorian et al.). Given inorganic phosphate’s significance in these two indispensable metabolic capacities involving cellular energy production and membrane formation, it fulfills an essential role in sustaining life at the cellular level.
Phospholipids exhibit a highly specialized molecular architecture that enables them to fulfill their crucial physiological roles. At the core of the phospholipid molecule lies a polar head group consisting of a phosphate moiety bound to a glycerol backbone. Extending outward from the glycerol are two nonpolar fatty acid tails. This asymmetrical, amphipathic configuration forms the basis for phospholipids’ unique property of self-assembling into bilayer structures when dispersed in an aqueous solution, a fundamental characteristic of their surrounding physiological environment both exterior and interior to cells (Zadoorian et al.). Due to their hydrophobic nature, the fatty acid tails aggregate and associate together inwardly between the two leaflets of the bilayer. Meanwhile, the hydrophilic head groups orient outwardly, interacting favorably with surrounding water molecules. Phospholipid bilayers can function as the basic building blocks of all biological membranes because of this ordered molecular configuration (Zhukov and Popov). For cells to separate metabolic processes and preserve the spatial structure required to sustain biochemical reactions and intracellular trafficking, these phospholipid membranes play a crucial role in separating intracellular regions and organelles from the exterior environment. The basic existence of life at the cellular level would be impossible without the selective permeability that phospholipid bilayers offer. Without this permeability, cells would not be able to compartmentalize functions and maintain structural integrity.
Multilamellar organelles represent a class of intracellular structures that are characterized by their complex, multilayered organizational architecture derived from phospholipid bilayers. These organelles, such as lysosomes, contain multiple phospholipid membranes that are spaced apart, separating intermembrane aqueous compartments within their volumetric space (Wilson et al.). Lysosomes function as the cell’s primary digestive vacuoles, containing hydrolytic enzymes that break biomacromolecules down into their constituent building blocks. As such, they provide an important example of a multilamellar organelle designed to carry out specialized metabolic functions. Other related organelles exhibiting multilayered membrane architecture include the Golgi apparatus and regions of the endoplasmic reticulum (ER). The Golgi apparatus and ER participate critically in modifying, processing, and transporting various cargo molecules essential for cell viability and homeostasis. Through their extensive, elaborated systems of internal phospholipid bilayers, these organelles are capable of developing highly optimized and complex three-dimensional interiors tailored for the specific metabolic activities that occur within their domains, such as macromolecular sorting and modifications (Wilson et al.). The intrinsic phospholipid bilayer structure common to all biological membranes thus facilitates the formation of these vital multilamellar organelles and endows them with elaborate, functionally advantageous architectural features adjusted for their roles in intracellular metabolic compartmentalization and flow of materials.
In conclusion, this introduction covered the fundamental phospholipid structure that permits the development of functioning biological membranes as well as the two important metabolic roles of inorganic phosphate. Gaining a deeper comprehension of these foundational ideas sets the stage for research on the dynamics of phospholipids in intricate organelle systems. Future sections will explore these organelle structures and dynamics in more depth.
Scientific Literacy #2: PXo Bodies
PXo bodies, new cell organelles emerged as a result of Drosophila melanogaster, which is among the commonly studied species in the kingdom Animalia. The chronic phosphate deprivation resulted in hyperproliferation of midgut epithelium. Chronic Pi starvation resulted in the down-regulation of PXo protein. PXo particularly marks non-canonical multilamellar organelles known as PXO bodies. This essay, therefore, explains the characteristics of PXo bodies, how PXo regulates levels of inorganic phosphate in the cytoplasm as well and how the formation of PXo bodies depends on the availability of inorganic phosphate.
PXo bodies are complex organelles that have characteristics of lipid and lysosome-rich structures. Based on Figure 2, PXo localizes to PXo bodies, and they are referred to as multilamellar organelles. In some plants, PXo ortholog localizes to the Golgi network as well as uncharacterized vesicles with exocytosis proposed as a Pi export mechanism. The GFP adds to the N-terminal of PXo while the HA adds to the C terminal of PXo, and they are markers of shape structures in fly midgut enriched with endogenous PXo (Suvvari, & Arigapudi, 2024). PXo bodies are recognized in progenitors. The characterization of PXo bodies shows that they are acidic, however, they do co-localize with endoplasmic reticulum markers, usually in proximity, to lysosome, endosome, mitochondria and exosome. Moreover, PXo bodies have one type of subcellular structures that are positively stained for the lipid dye and Nile Red. Another characteristic is that they are in proximity and never co-localize with cis-, medial-, and trans-cisternae markers such as GMAP and MAnII for the Golgi body.
PXo regulates cytosolic Pi in PXo bodies. PXo mediates Pi transport from the cytosol into PXo bodies due to its predominant localization. Figure 3 shows levels of cytosolic Pi with a FRET-based Pi sensor known as cpFLIPPi-6.4. The FLIPPi’s FRET ratio is regarded as a fluorescence ratio of CPVenus versus CFP and this correlates to the cytosolic pi levels. PXo knockdown minimizes the ratio of FRET while progenitors consisting of PXo overexpression show an increased ratio of FRET (Xu et al., 2023). However, in progenitors, the PXo knockdown has no impact on FRET ratios, and this is a result of low PXo abundance and PXo bodies in progenitors. Therefore, from the figure, it is evident that PXo transports cytosolic Pi to PXo bodies, and this is essential in restricting levels of cytosolic Pi.
We can tell that the formation of PXO bodies entirely depends on the availability of inorganic phosphate by examining their morphology and abundance in Pi starvation. PXo bodies are usually smaller following MSF10 knockdown-induced Pi starvation. Following Pi starvation and based on Figure 4, endogenous PXo mRNA levels reduce while the residual endogenous puncta get engulfed by large lysosomes as well as induced autophagosomes. Moreover, from Figure 4, the PFA-induced degradation of PXO bodies and GFP-PXo are rescued by lysosome inhibitors Bafilomycin A1 and not MG132 inhibitors. The expression as well as the staining of the SPX-truncated PXo version are not impacted by PFA (Xu et al., 2023). Thus, we conclude that the formation of PXo bodies depends on the availability of Pi for biogenesis and encounters SPX-dependent lysosomal degradation after Pi starvation.
A decrease in phosphate levels results in alterations in phospholipid compositions as well as metabolism. From the pie charts in Figure 5, it is clear that there are different levels of phospholipids from the normal food and PFA.
Conclusion
The data convinces me that PXo bodies form distinct organelles with unique biochemical functions in the cell. This is because the formation of these distinct organelles entirely depends on particular characteristics within the cell.
All References Used:
Suvvari, T.K. & Arigapudi, N., 2024. Unravelling the mystery of PXo bodies: Insights into a newly identified cell organelle. Science Progress, 107(1), p.00368504241234242. https://doi.org/10.1177/00368504241234242
Xu, C., Xu, J., Tang, H.W., Ericsson, M., Weng, J.H., DiRusso, J., Hu, Y., Ma, W., Asara, J.M. & Perrimon, N., 2023. A phosphate-sensing organelle regulates phosphate and tissue homeostasis. Nature, 617(7962), pp.798-806. https://doi.org/10.1038/s41586-023-06039-y
Dunn, Jacob, and Michael H. Grider. “Physiology, Adenosine Triphosphate.” Nih.gov, StatPearls Publishing, 13 Feb. 2023, www.ncbi.nlm.nih.gov/books/NBK553175/. Accessed 12 Mar. 2024.
Wilson, Zachary N., et al. “Mitochondrial-Derived Compartments Are Multilamellar Domains That Encase Membrane Cargo and Cytosol.” BioRxiv (Cold Spring Harbor Laboratory), Cold Spring Harbor Laboratory, July 2023, https://doi.org/10.1101/2023.07.07.548169. Accessed 12 Mar. 2024.
Zadoorian, A., et al. “Lipid Droplet Biogenesis and Functions in Health and Disease.” Nature Reviews Endocrinology, vol. 19, no. 8, Nature Portfolio, May 2023, pp. 443–59, https://doi.org/10.1038/s41574-023-00845-0. Accessed 12 Mar. 2024.
‌Zhukov, Anatoly, and Valery Popov. “Eukaryotic Cell Membranes: Structure, Composition, Research Methods and Computational Modelling.” International Journal of Molecular Sciences, vol. 24, no. 13, Multidisciplinary Digital Publishing Institute, July 2023, pp. 11226–26, https://doi.org/10.3390/ijms241311226. Accessed 12 Mar. 2024.
⭐️ End-Of-Term Reflection Post:
Cell Biology has 100% been my favorite class this semester. From the challenging course work, to the weekly packback discussions, this class has challenged me as an overall student and allowed me to gain deeper knowledge in my major of Biomedical science. One specific thing I learned throughout the course this semester that helped me grow as a student, and also helped me connect to other coursework from different classes was the SmartBio assignment Lesson 3 DNA Packaging. This specific lesson helped me with my understanding of my Genetics class as well as this class. I picked this specific lesson to speak about because I remember it being super in depth about the topic and I almost immediately tied that knowledge into the coursework from my Genetics class as well. This class has taught me a lot about Cell Biology and I cannot wait to further my education at ODU.